Crystal clear
Almost every radio has a crystal oscillator — here's how they work.
October 1, 2009
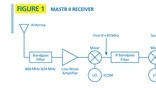
Radio receivers and transmitters both require a precise frequency reference, and this reference almost always is provided by a crystal oscillator. A crystal oscillator is an electronic circuit that uses the mechanical resonance of a vibrating crystal to generate a sinusoidal electronic signal at a very precise frequency. The translation by certain materials of a a mechanical impulse to an electrical oscillation is called the piezoelectric effect. The most common type of piezoelectric resonator is the quartz crystal and electronic circuits designed around them are called crystal oscillators.
Piezoelectricity was discovered by Jacques and Pierre Curie in 1880. The first crystal-controlled oscillator was constructed at Bell Laboratories in 1917 by Alexander Nicholson using a crystal of Rochelle salt. Walter Cady, a professor at Wesleyan University, built the first quartz crystal oscillator in 1921. By 1926, quartz crystals were used to control the frequency of AM radio broadcast transmitters and were widely used by amateur radio operators.
To illustrate the application of crystal oscillators to land-mobile radio, we will consider the Harris 800 MHz MASTR II repeater. The MASTR II repeater was manufactured from the mid-1980s through 1993. It is still used in public safety and private LMR systems, but 800 MHz rebanding in the United States caused many MASTR IIs to be replaced because the crystal oscillator in the receiver was no longer supported and no reliable substitute was readily available. Modern receivers do not have this problem because a single frequency oscillator (usually 10 MHz) is used in all receivers and the tuned frequency is generated by a frequency synthesizer using the the fixed oscillator as a reference. Although the MASTR II is obsolete, the problem with the receiver oscillator reveals the temperature and aging variations that apply at some level to all crystal oscillators.
Virtually all LMR receivers, including the MASTR II, employ a superheterodyne receiver that requires a particularly accurate and pure local oscillator (LO). In the MASTR II receiver, two local oscillators are used with mixers to step down the radio frequency signal from a frequency in the receive band (806-824 MHz) to each of two intermediate frequencies, where effective filtering and demodulation can take place. The first LO is generated by an Integrated Circuit Oscillator Module (ICOM) — not to be confused with the radio manufacturer of the same name. The ICOM uses a precisely cut quartz crystal and a matched temperature compensation circuit to generate the LO frequency. A MASTR II repeater cannot be retuned without replacing the ICOM or replacing the crystal in the ICOM.
A block diagram of the MASTR II receiver is shown in Figure 1. Note that the ICOM is the subassembly that generates the first LO frequency. In the MASTR II receiver, the LO is 45 MHz below the receive frequency.
The ICOM is a metal package that consists of a single quartz crystal and associated circuitry that generates a pure sine wave at the desired frequency. The precise physical dimensions of the quartz crystal determine its frequency. When a voltage is applied across the quartz crystal, the crystal will flex at its resonant frequency.
Due to internal losses within the crystal, these small oscillations would normally decay and at some point oscillation would stop. To ensure the oscillations persist, an external amplifier is used to overcome the losses and reinforce the oscillation. The term given to the combined crystal and amplifier circuit is the oscillator. Typically, the resonant frequency of the crystal is lower than the desired LO frequency and a multiplier circuit is used to raise the crystal frequency to the LO frequency. For example, the MASTR II receiver uses a multiplier factor of 48 and the multiplier circuit is external to the ICOM. Because the MASTR II LO is 45 MHz below the radio frequency (806.0125-823.9875 MHz) and the multiplier factor is 48, they receive ICOM crystal frequencies varying from 15.854427 MHz to 16.228906 MHz. Because the multiplier is fixed, a different crystal is needed for each of the more than 800 frequencies between 806.0125 and 823.9875 MHz.
The oscillator’s developed voltage is a sine wave whose frequency is directly related to the physical dimensions (resonant frequency) of the crystal mass. Changing the oscillator’s frequency requires one to make dimensional changes to the crystal mass. That is, crystals must be custom tuned by cutting or grinding to exact dimensions and tolerances to achieve a highly accurate desired frequency.
Although crystals once ground are, in a general sense, dimensionally stable, their instantaneous resonant frequency is affected by environmental changes, especially temperature.
Temperature control and temperature compensation. Crystal stability can be improved through the use of thermostatically controlled ovens (where the temperature is set to an artificially high, precisely-controlled level) or with electronic compensation circuitry whose temperature characteristics are selected to be exactly opposite of those of the crystal. Oven-controlled oscillators are denoted by the acronym OCXO while temperature-controlled oscillators are denoted by the acronym TCXO. The ICOM is a TCXO. When a crystal and compensation network are properly paired, the oscillator’s frequency is, for all practical purposes, independent of temperature variations.
The ICOM in the MASTR II base station incorporates three critical elements: a precisely tuned (ground-to-frequency) crystal, an amplifier and a custom-optimized automatic temperature compensation circuit.
The temperature compensation circuit is designed to maintain the ICOM frequency within precisely-established FCC requirements over a temperature range of -30 to +85° C.
Due to structural differences between crystals and the grinding process itself, each temperature compensation network for 800 MHz ICOMs used in MASTR II base stations was individually optimized specifically for the installed crystal.
Temperature compensation circuits operate at two extremes: low temperatures and high temperatures. At the low end, temperature compensation circuits activate at temperatures below 0° C (32° F). When the temperature drops below 0°C, the circuit is activated. As the temperature decreases, an equivalent resistance decreases and the compensation voltage increases. An increase in compensation voltage decreases the capacitance of the varactor in the oscillator, thereby raising the output frequency of the ICOM.
At the high end, temperature compensation circuits for the 800 MHz ICOM operate at temperatures above +55° C (131° F). When the temperature rises above 55° C, the first branch of the compensation circuit activates. At temperatures above 70° C, the second branch of the circuit activates so that both branches are now operating simultaneously. At temperatures above each of these activation points, the equivalent resistance decreases, thereby decreasing the compensation voltage. The decrease in compensation voltage increases the capacitance of the varactor, thereby lowering the output frequency of the ICOM.
The ICOM has a specified frequency accuracy of 1 part per million (ppm) over its specified temperature range of -30° C to +85° C, and 0.2 ppm at an ambient temperature of 27.5°C (81.5° F).
Frequency drift in crystal oscillators and the importance of aging. A characteristic of crystals is that they age over time and some of this aging occurs after the crystal is put into service. Aging causes frequency drift in the ICOM crystal, which is multiplied by the 48X multiplier circuit. While each crystal is unique in the way it ages, one can count on some drift from all crystals. It is generally understood in the industry that the original manufacturer, General Electric, aged ICOMs for 60 days. This aging process included temperature cycling of the ICOM while it was under operating conditions. Thus, the crystal and its matched temperature compensation network were aged and tested in the factory for an extended period to minimize drift once in the field. Once a non-defective crystal is fully aged, it is stable in time and temperature, provided the temperature compensation circuit is properly matched to the crystal. Like a good wine, no crystal oscillator should be sold before its time.
Jay Jacobsmeyer is president of Pericle Communications Co., a consulting engineering firm located in Colorado Springs, Colo. He holds bachelor’s and master’s degrees in electrical engineering from Virginia Tech and Cornell University, respectively, and has more than 25 years experience as a radio-frequency engineer.