Designing microwave radio links
They remain the preferred backhaul choice for public safety radio networks
April 1, 2008
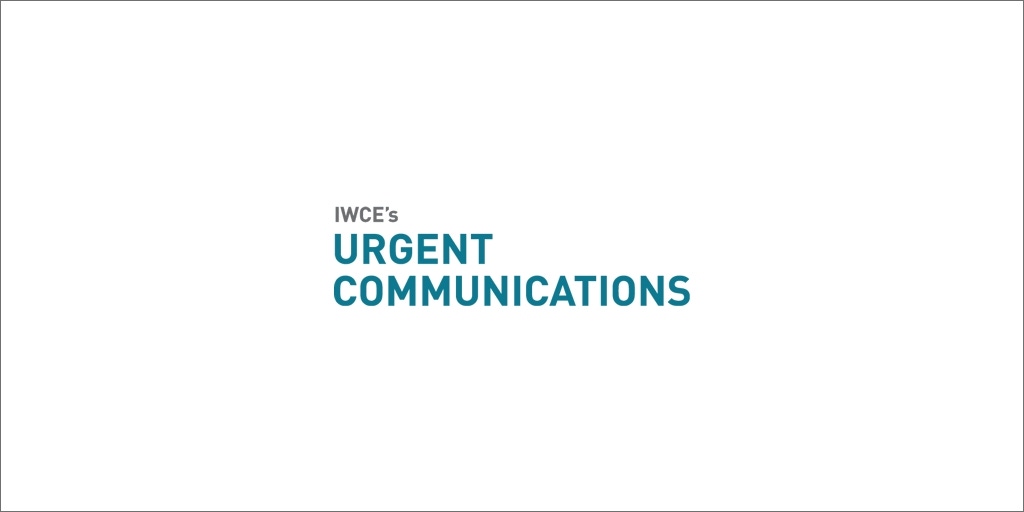
The golden age of point-to-point microwave was the 1960s, when nearly all long-distance telephone calls and all network television signals traveled over the medium. Eventually, fiber optic cable replaced microwave for most telephone calls, and satellite took over for television.
Today, cellular radio networks rely primarily on landline T-1s for backhaul, but most public safety radio networks use microwave radio for this purpose. Microwave is preferred by public safety because the recurring costs are lower than leased T-1s, and reliability can be higher than landline networks. As an old AT&T microwave engineer once said to me, a landline can be cut at an infinite number of points, but a microwave link can fail at only two spots. Modern microwave links often are organized into self-healing rings with equipment and path redundancy to ensure a single equipment failure does not cause a network failure.
Microwave paths are quite different from land mobile radio paths. Unlike mobile radio, microwave radios are always stationary, and every effort is made to ensure the antennas are line-of-sight. The dominant impairment should be free space loss, and fading occurs not from scattering but from atmospheric effects.
Microwave paths must have an extremely high availability (greater than 99.995%) and low bit-error rate (less than 10?9) in order to be effective. Bandwidths of microwave channels are much wider than most mobile radio channels, usually on the order of 30 MHz, with bit rates as high as 155 Mb/s. To achieve these bit rates, higher-order signal constellations such as 64-QAM are used with trellis-coded modulation.
In the U.S., the FCC allocates spectrum for microwave point-to-point links, and frequency coordination is required as part of the license application. Antenna gain and polarization are used to pack frequencies very tightly. Depending on the band, high-performance antennas with reduced sidelobes may be required to mitigate interference to other systems. Popular microwave bands include the 2, 4, 4.9, 6, 10, 12, 18 and 23 GHz licensed bands and the 2.4 and 5.8 GHz unlicensed bands.
This article describes the process for designing microwave links, which involves picking the sites, plotting the path profile, predicting the path loss and calculating the path availability.
Site selection
In LMR networks, microwave radio sites are usually co-located with the LMR tower and the tower height is used to ensure a line-of-sight path. Sometimes an intermediate relay is necessary when terrain or buildings block the path.
Path profile
After the prospective sites are chosen, the path profile is the first step to ensuring the feasibility of the path. The profile indicates whether the path is free of obstructions. Because the atmosphere has a refractive index gradient that tends to bend radio waves back toward the earth, the radio horizon is usually greater than the optical horizon. The standard propagation model assumes an equivalent earth radius equal to 4/3 the actual radius. To capture this effect on the path profile, a 4/3 earth profile is used.
Before the advent of personal computers, the designer plotted the profile on special graph paper. Today, the computer creates the 4/3 earth profile when it plots the terrain profile, or equivalently, the terrain is plotted on a flat earth and the path is curved to show the effect of a 4/3 earth. Sometimes anomalous atmospheric conditions occur and create the equivalent of a smaller earth radius. For this reason, some paths also are plotted assuming a worst-case • earth radius.
Terrain usually is the dominant factor in the profile. Digital terrain databases are used to save the time and trouble of extracting elevations from topographic maps. Generally speaking, a high-resolution database such as the 30-meter database produced by the U.S. Geological Survey is preferred.
Buildings and foliage also can obstruct the path, and these obstructions usually are not indicated on digital terrain databases. Therefore, a thorough site survey is required before the path is selected for construction. A typical path profile is shown in Figure 1.
Path loss prediction
A simplified link budget can be calculated using Equation 1, while the required signal amplitude for a particular path-availability can be found via Equation 2.
The lack of sufficient clearance above terrain obstacles will result in attenuation of the radio signal. Pure line-of-sight is not sufficient because all radio waves cast shadows, and a sufficient clearance above obstacles is required to ensure there is no diffraction loss. Clearance above terrain is measured in terms of the Fresnel zone radius, or Fn, where n = 1, 2, 3, etc. From diffraction theory, we can calculate the radius of the nth Fresnel zone using Equation 3.
Usually, we are concerned only with the first Fresnel radius, F1. The path clearance must be at least 0.6 F1 to ensure negligible attenuation. At grazing (0.0 F1), the attenuation can be as high as 15 dB, depending on the shape of the obstacle and its conductivity.
The Fresnel radius reaches its maximum level at the center of the path, as shown in Figure 1. In the late 1950s, Ken Bullington published a nomogram that predicted the obstacle diffraction loss as a function of clearance. This nomogram is useful, but most designers simplify the problem to creating adequate clearance so that the predicted diffraction loss is zero, meaning that the clearance must be at least 0.6 F1.
Path availability
The path availability (also called link reliability) is the percentage of time that the received signal is above the required threshold, Preq. It is sometimes expressed as the expected minutes of outage per year. The path availability is a function of the radio frequency, diversity (if any), fade margin, path length, and local climate. The International Telecommunication Union publishes reports with empirical models of required fade margin for different parts of the world. Designers use these reports to ensure adequate fade margins. A typical design requirement is 99.995%, which is equal to an expected outage of 26 minutes per year.
Jay Jacobsmeyer is president of Pericle Communications Co., a consulting engineering firm in Colorado Springs, Colo. He holds BS and MS degrees in electrical engineering from Virginia Tech and Cornell University, respectively, and has more than 25 years of experience as a radio frequency engineer.
Equation 1
Pr = Pt + Gt – Lfs – Lo – Lc + Gr
where Pr = received signal power in dBm
Pt = transmit power in dBm
Gt = transmit antenna gain in dBi
Lfs = free space loss in dB = 22 + 20 log10(d/λ), where d is the path length and λ is the radio carrier wavelength
Lo = all other path losses including diffraction loss and rain attenuation
Lc = cable and waveguide losses
Gr = receive antenna gain in dBi
Equation 2
Preq = -174 + 10 log10(Bn) + NF + C/Nreq + FM
where Bn = equivalent noise bandwidth of the receiver in Hz
NF = receiver noise figure in dB
C/Nreq = required carrier-to-noise ratio for a particular bit-error rate
FM = fade margin to account for fading and rain attenuation, usually 25 to 40 dB
Equation 3
where λ is the wavelength of the radio carrier
d1 = the distance from the first end point to the point of interest
d2 = the distance from the second end point to the point of interest
Fn = √ nλ d1d2/d1+d2
References
Ken Bullington, “Radio Propagation Fundamentals,” Bell System Technical Journal, vol. 36, no.3, 1957.
International Telecommunications Union, TU-R P.530, “Propagation data and prediction methods required for the design of terrestrial line-of-sight systems,” Feb 2007.